TL;DR: Superconductors and quantum computing hold the key to creating ultra-efficient power systems and blazing-fast computers, promising a technological revolution that could reshape modern life.
The Quiet Revolution: Why Superconductors and Quantum Computing Matter
Superconductors and quantum computing often sound like distant, ultra-technical fields. Yet behind these terms lie the next big leaps in how we generate power, process information, and even understand reality.
Picture a world where electricity flows with zero resistance, meaning no energy lost as heat. That’s the promise of superconductors, materials that, when cooled to very low temperatures, let electrical current glide without friction. It’s like a highway with no speed limits and no traffic jams.
Meanwhile, quantum computing is all about harnessing the quirky rules of quantum mechanics—nature at its smallest scale—to solve problems that stump even the most powerful supercomputers. From cracking complex scientific puzzles to optimizing massive logistics, the potential is enormous.
Together, these two breakthroughs promise to revolutionize technology by supercharging computing speeds, slashing energy consumption, and enabling scientific discoveries that once seemed impossible. It’s not just lab curiosity—global tech giants and startups alike are racing to build the future on these foundations.
Superconductors 101: A Gateway to Effortless Electricity
Many people associate electricity with heat. Standard wires and cables offer some resistance, which wastes energy as they transport current. This is why you might feel warmth in a phone charger after it’s been plugged in a while.
But superconductors eliminate that inefficiency. Below a certain temperature called the critical temperature, a superconducting material conducts electricity without losing any energy as heat. Imagine if your smartphone never heated up, or if overhead power lines could deliver electricity from a power plant to your home with almost zero loss.
When Zero Resistance Becomes Reality
Electrical resistance arises when electrons in a wire collide with atoms, losing energy as heat. In a superconducting state, electrons move in cooper pairs—they pair up in a dance that makes them immune to such collisions.
It’s as if the electrons team up, forming a “superhighway lane” through which they glide freely. While these effects often require extreme cold (–196 °C / –321 °F or lower) with liquid nitrogen or helium, new high-temperature superconductors continue to push the boundaries of feasibility.
The Magic of Magnetic Levitation and Beyond
One of the most eye-catching demonstrations of superconductivity is magnetic levitation, where magnets float eerily above a supercooled superconductor. This happens because superconductors expel magnetic fields, a phenomenon known as the Meissner effect.
Beyond scientific demonstrations, magnetic levitation can pave the way for frictionless trains, advanced imaging in hospitals via MRI (Magnetic Resonance Imaging), and more efficient motors. The ability to harness magnetism and electricity with negligible losses opens up engineering possibilities far beyond what ordinary conductors allow.
Diagram: How Superconductivity Splits Into Multiple Applications

Diagram: Multiple paths of superconductivity usage, from power and transport to medical applications.
Quantum Mechanics in a Nutshell
Quantum mechanics is the branch of physics dealing with the smallest particles: electrons, photons, and other building blocks of the universe. Unlike everyday objects, these particles follow counterintuitive rules—like existing in multiple states at once (superposition) or “talking” to each other across distances (entanglement).
Though it sounds bizarre, these quantum behaviors have been experimentally confirmed for decades. They underpin technologies like lasers, transistors, and atomic clocks. Quantum computing takes this a step further by using these weird rules for processing information in profoundly more powerful ways than classical computers can.
Quantum Computing: Why It’s a Game-Changer
Traditional computers store data as bits, which can represent 0 or 1. Quantum computers use qubits—which can be 0, 1, or both at the same time (superposition). It’s akin to flipping two coins and allowing them to exist in all possible heads/tails combinations simultaneously before you even look at them.
This opens the door for massive parallelism. A quantum processor can explore countless paths in a single computational step, giving quantum computers the edge in solving certain complex tasks—like simulating molecules for new drug discoveries or cracking sophisticated encryption.
From Nanoparticles to Medical Breakthroughs
Imagine simulating the interactions of molecules with near-perfect accuracy. That capability could transform pharmaceutical research, letting scientists pinpoint the best drug formulations instantly instead of relying on trial and error. Or think about fine-tuning materials for greener energy storage and carbon capture.
Quantum computing also promises leaps in machine learning, risk analysis, and logistics. For large-scale optimization problems—like scheduling flights for thousands of planes every day—quantum computers can find solutions that reduce delays, cut costs, and save fuel. This computational power extends to finance, climate modeling, and any domain needing multi-variable optimization.
Superconductors: The Foundation for Quantum Computing
So where do superconductors come in? Qubits are extremely delicate. They lose their quantum state (or decohere) when exposed to everyday noise—stray electromagnetic fields, heat, or even cosmic rays. Superconducting circuits minimize this noise by letting electrical current flow without collisions and with tight temperature control.
Josephson Junctions: The Heart of Superconducting Qubits
A Josephson junction is a tiny device formed by two superconductors separated by a thin insulating barrier. Electrons tunnel quantum-mechanically across the barrier, enabling precise control over the qubit’s energy states. These junctions form the building blocks of many quantum computers, allowing engineers to tweak the qubit’s frequency and keep it coherent long enough to perform calculations.
When cooled near absolute zero (around –273 °C / –459 °F), superconducting qubits can remain coherent for microseconds—long enough to do hundreds or thousands of quantum operations. That might sound fleeting, but in the quantum realm, it’s quite an achievement.
Diagram: How Superconductors Enable Quantum Computing
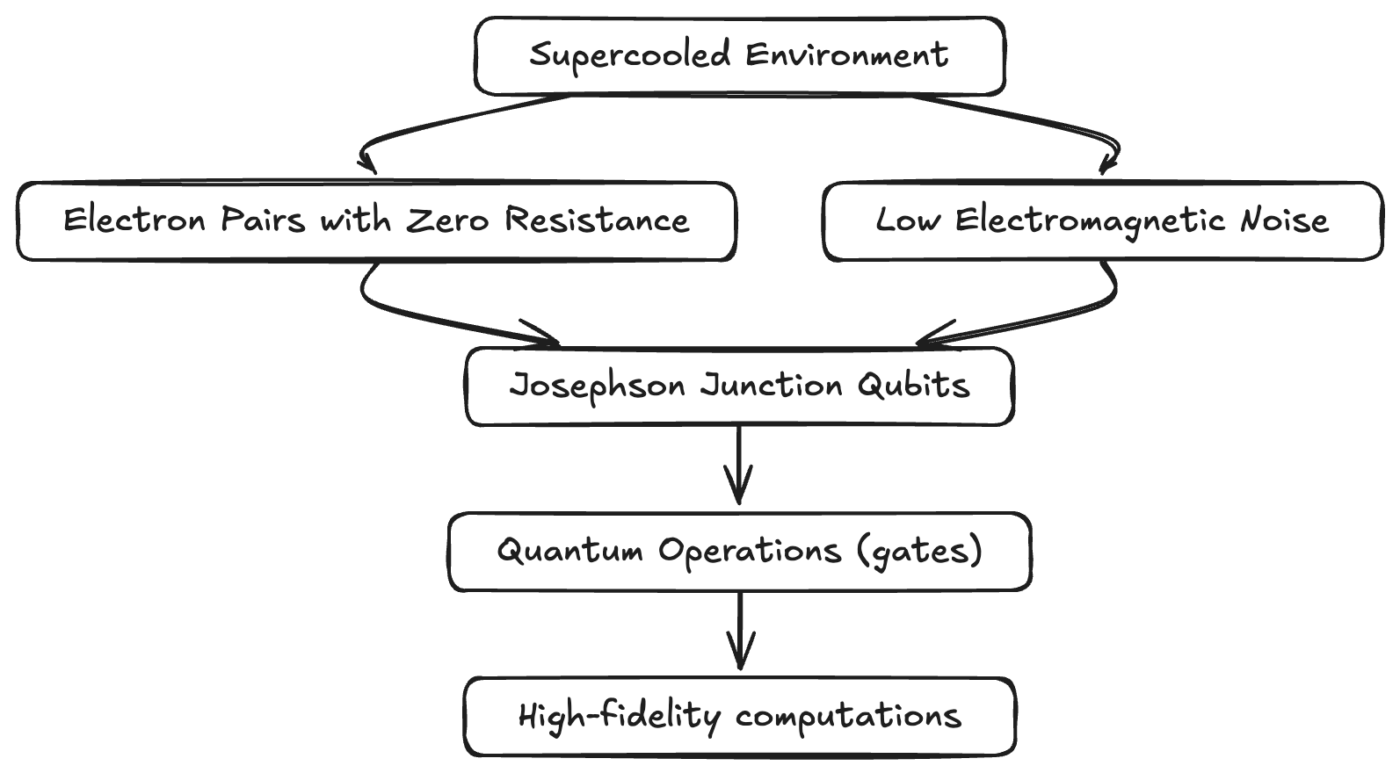
Diagram: Illustrates how superconductors allow near-noiseless conduction, enabling stable qubits for quantum operations.
Envisioning Revolutionary Impacts
Quantum computing and superconductors may seem futuristic, but the revolution is already unfolding. Let’s explore just a few fields that stand to benefit:
1. Energy and Power Grid Efficiency
With superconducting cables, large cities could receive power from distant sources with minimal energy loss. This might dramatically cut carbon emissions, as power plants would waste less fuel. Supergrid initiatives could integrate renewables—like wind or solar—on a continental scale.
Add quantum computing to the mix, and you can optimize energy distribution in real-time. Picture a grid that instantly reroutes electricity to where it’s needed, guided by algorithms that factor in weather, demand, and price signals.
2. Ultra-Fast Computing for AI
AI often demands huge computational power. Quantum computers, in tandem with superconducting technologies, could address machine learning tasks at unprecedented scales. Training a large language model or an advanced image-recognition system might drop from weeks to hours, enabling faster breakthroughs in healthcare diagnostics, climate modeling, and scientific research.
3. Pharmaceutical and Material Innovations
As mentioned, quantum computers can model complex molecules precisely. That’s a boon for drug discovery—cutting years of research into months, saving billions of dollars. Superconducting labs are also working on exotic materials (like high-temperature superconductors) that could revolutionize everything from efficient motors to floating trains (maglev).
4. Cybersecurity and Encryption
Quantum computers can potentially crack current encryption methods. This threat spurs development of quantum-safe encryption to protect data. On the flipside, quantum computing can also create perfectly secure communication through quantum key distribution (QKD). Meanwhile, superconducting quantum bits can help demonstrate these encryption protocols in real-world tests.
Myth-Busting: Superconductors and Quantum Hype
Myth: We Already Have Room-Temperature Superconductors
Reality: Despite headlines, a true stable, room-temperature superconductor is still elusive. Researchers occasionally report breakthroughs that turn out to require extremely high pressures or yield questionable reproducibility. Most current superconductors operate at cryogenic temperatures.
Myth: Quantum Computers Will Replace All Computers
Reality: Classical computers remain better for everyday tasks like browsing, word processing, or gaming. Quantum computers shine in specialized areas, like large-scale optimization or molecular simulations, where parallel quantum states can drastically reduce computation time.
Myth: Quantum Computing Is Sci-Fi, Decades Away
Reality: Though large-scale quantum computers are still in development, functional quantum processors already exist with dozens or even hundreds of qubits. Giant tech firms and startups are racing to scale up. We may see real-world quantum applications in the next few years—particularly in fields like finance, materials science, and cryptography.
Quantum vs. Classical: Understanding the Core Difference
Conventional computers operate step-by-step, evaluating each possibility in turn. Quantum computers, by contrast, evaluate many possibilities simultaneously thanks to superposition. This allows them to approach certain problems in ways that blow classical algorithms out of the water.
However, reading the result is tricky. Measuring a qubit “collapses” it to a definite 0 or 1. Much of quantum computing research focuses on how to carefully craft calculations so that when we measure, the correct answer emerges with high probability. Superconductors help minimize external noise that can ruin this delicate process.
Seeing the Future: Challenges and Triumphs Ahead
Scaling Up Qubit Counts
The biggest immediate challenge is scaling from tens or hundreds of qubits to thousands or millions, a threshold for solving truly “impossible” problems. Handling so many qubits means building massive cryogenic setups, preventing heat from creeping in, and ensuring signals are pure.
Error Correction and Stability
Quantum systems are prone to decoherence and errors. Scientists use quantum error correction methods to spread information across multiple qubits so a single error doesn’t corrupt the entire computation. This technique demands extra qubits and complex control electronics—both of which rely on stable superconducting architectures.
Industrial and Commercial Interest
Financial institutions, pharmaceutical companies, and tech giants are already investing heavily. They see quantum computing as a chance to leapfrog rivals. Government agencies also view quantum computing as a strategic priority, funding large research programs to stay at the forefront of cryptography and technological competitiveness.
Earth-Sized Comparisons
If the Earth were the size of a basketball, a single electron would be smaller than a dust speck on its surface. Quantum computing deals with phenomena at scales even smaller than that electron’s interactions. Meanwhile, if all the power lines in a major city had zero resistance—like superconductors do—the city’s wasted energy could be cut drastically, akin to saving enough energy to power thousands of homes continuously. These comparisons highlight just how big an impact these tiny-scale physics can have on our day-to-day lives.
Potential Breakthroughs: A Glimpse at Tomorrow
- Global Superconducting Grids: Seamlessly link solar farms in deserts, wind turbines offshore, and cities worldwide.
- Instant Molecular Simulations: Rapid drug design, potentially curing diseases much faster than we can now.
- Commercial Fusion Reactors: High-power superconducting magnets help contain plasma at the core of fusion experiments, bringing us closer to near-limitless clean energy.
- Next-Gen Computers: Hybrid machines that combine classical and quantum processors, bridging routine tasks with high-powered quantum calculations when needed.
- Space Exploration: Lightweight superconducting systems for deep space missions could enhance propulsion, communication, and scientific instruments.
FAQ Section
How cold do superconductors need to be?
It varies. Some, like niobium-titanium, need temperatures near 4 K (–269 °C / –452 °F). Others, called high-temperature superconductors, can function around 77 K (–196 °C / –321 °F) using liquid nitrogen. True “room-temperature” superconductors remain a goal, but not a current reality.
Are there alternative qubit platforms besides superconductors?
Yes, some groups use ion traps (captured charged atoms), photonic qubits (light particles), or spin qubits in silicon. Each has pros and cons, but superconducting qubits are popular due to their established fabrication techniques and decent coherence times.
Could quantum computing risk data breaches?
Potentially. A powerful quantum computer might break many current encryption methods. That’s why “post-quantum cryptography” and quantum-safe solutions are in development. It’s a race between quantum hackers and cryptographers.
Why do we need vacuum or cryogenic conditions for quantum computing?
Qubits are extremely sensitive to any interference. Thermal energy, electromagnetic noise, and even cosmic rays can disrupt quantum states. Cooling to cryogenic temperatures quiets the environment, allowing quantum effects to dominate.
Do superconductors work in everyday electronics like smartphones or laptops?
For now, no. The need for cryogenic cooling is a practical barrier to everyday consumer devices. Future breakthroughs in high-temperature superconductors could one day bring near-zero resistance to everyday gadgets.
Myth-Busting Revisited
Myth: Superconductors are only for big labs
Reality: While large-scale uses in labs are prominent, superconducting technologies are already in real-life devices like MRIs. Innovations in miniaturization and cooling might see broader adoption in power grids or specialized electronics.
Myth: Quantum computers will solve every problem instantly
Reality: They excel at certain classes of problems, particularly ones with massive parallel computations or tricky optimization. But for everyday tasks—like streaming movies or browsing the web—classical systems remain more efficient.
A Future Shaped by Quantum-Enabled Superconductors
From hyper-efficient power grids to lightning-fast data centers, superconductors could cut global energy waste and revolutionize everything from mass transit to medical imaging. Layer on top the coming wave of quantum computing—able to tackle intractable problems—and the stage is set for a new technological era.
The synergy of these fields might produce breakthroughs that feel like science fiction today. Real-time weather manipulation or near-instant solution of protein-folding problems could become routine tasks for a quantum supercomputer plugged into a superconductive network. Although challenges remain, the promise of these technologies is real and increasingly within reach. The 21st century may see them become integral to daily life in ways we’re only beginning to imagine.
Read More
- Superconductivity: A Very Short Introduction (Amazon)
- Quantum Computing for Everyone (Amazon)
- The Future of Fusion Energy (Amazon)
- Official IBM Quantum Computing Site
- MIT’s Technology Review – Superconductor Research
These resources will provide deeper insights into how superconductors and quantum computing have evolved—and how they’re set to transform technology as we know it.